Operation modes
Most MFM experiments are performed under ambient conditions. Under these conditions, a dual passage method is typically used [1, 2]: In the first scan the intermittent contact mode is used to map the topography of the sample. The latter is then used to scan the magnetic signal with the tip lifted off the surface of the sample. A serious drawback of this technique is being incompatible with operation under vacuum conditions. Moreover, topographical features can influence the signal recorded in the second passage scan, when the tip is lifted off the sample surface. This is because the magnetic force is non-local and strongly depends on the tip-sample distance. Topography induced distance changes can thus lead to the convolution of the topography with the measured “magnetic” signal.
For this reason we prefer to scan parallel to the average sample slope at constant average height (which is of course possible only for flat samples). In the past this was typically done with a stopped z-feeback or with a slow z-feedback to keep the average frequency shift signal at a chosen setpoint value.
We have recently developed three single passage MFM/KPFM operation modes suitable for vacuum operation that make use of multi-frequency techniques: The first method [3] mechanically excites the cantilever oscillation simultaneously on its first and second resonances to map the magnetic forces and the topography via short-ranged van der Waals forces. Because these are short-ranged, the tip needs to follow the local topography with nanometer precision. Scanning at an arbitrary constant average tip-sample distance is thus not possible. The second [4] and third [5] operation methods also use the first cantilever resonance to map the magnetic forces, but excite the cantilever via an oscillatory tip-sample bias either at half of the resonance frequency of the second cantilever oscillation mode [4] or at a much lower frequency, typically around 1kHz to obtain a frequency-modulation of the fundamental mode cantilever oscillation [5] (see figure below and video abstract click here). The second mode oscillation amplitude or the second side-band amplitudes, respectively, are then a measure for the tip-sample capacity, which can be kept constant locally or in average by the z-feedback. The frequency-modulated capacitive tip-sample distance control mode [5] allows keeping the tip-sample distance constant even when the applied magnetic field is increased from 0 to 7T. Under these conditions, the cantilever bends several tens of nanometers, the frequency shifts by tens of Hertz, and the cantilever quality factor changes by more than ten percent. Nevertheless, the tip-sample distance can be kept constant with a precision of about half a nanometer.
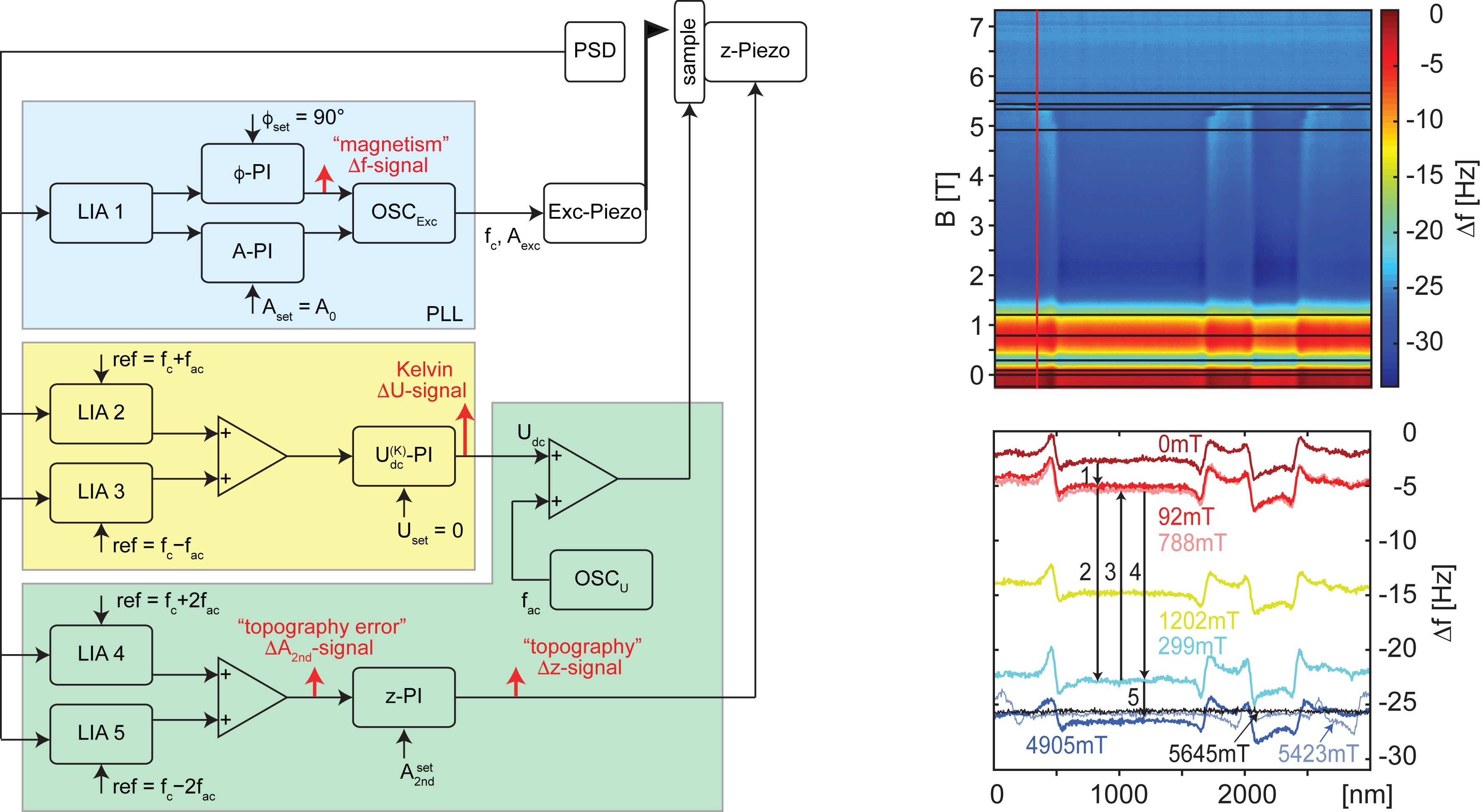
References
[1] Hosaka S, Kikukawa A, Honda Y and Koyanagi H. (1992) Jpn. J. Appl. Phys. 31, L908
[2] Giles R, Cleveland J P, Manne S, Hansma PK, Drake B, Maivald P, Boles C, Gurley J and Elings V.
(1993) Appl. Phys. Lett. 63, 617
[3] Schwenk J, Marioni MA, Romer S, Joshi NR and Hug HJ. (2014) Appl. Phys. Lett. 104, 112412
[4] Schwenk J, Zhao X, Bacani M, Marioni MA, Romer S and Hug HJ. (2015) Appl. Phys. Lett. 107, 132407
[5] Zhao X, Schwenk J, Mandru AO, Penedo M, J, Bacani M, Marioni MA and Hug HJ. (2018) New J. Phys. 20, 013018
-
Share